This theorem puts a limit on the amount of information that can be communicated over a channel with a given bandwidth and amount of noise. The famous equation for this is: C/B = Log2 (1+SNR)
(C) is the maximum achievable channel capacity, (B) is the bandwidth, and (SNR) is the ratio of signal power to noise power.
In the context of fibre capacities, this limit is crucial because it defines the theoretical upper bound on the data rate that can be transmitted over a fibre optic cable without errors, given the presence of noise. This limit is often referred to as the “Shannon limit.”
Subscribe today for free
As the signal-to-noise ratio (SNR) increases, the channel capacity increases, but the relationship is logarithmic, meaning that doubling the SNR value only results in a modest increase in capacity. In practical terms, this means that as we try to push more data through fibre optic cables, we encounter diminishing returns. This has led to innovations in modulation techniques and error-correcting codes to approach this theoretical limit as closely as possible.
In the late 1990s and early 2000s, we increased fibre capacities mainly by expanding transmission to a wider spectrum of fibre. In the favoured C-band of the fibre, which offers the lowest attenuation or “loss per kilometre,” we expanded from 3.2 THz to 4.8 THz by enhancing erbium-doped fibre amplifiers (EDFAs) and transceiver tunability.
The introduction of coherent optical technology in the 2010 timeframe increased fibre capacity with more bits per symbol enabled by polarisation multiplexing and quadrature amplitude modulation (QAM) that leverages both phase and amplitude. Today PM-64 QAM modulation’s 12 bits per symbol is the most economical maximum number of bits per symbol.
Increasing bits per symbol could enhance fibre capacity and spectral efficiency a little further, but it also poses challenges in terms of reach, noise, and power consumption. These factors need to be balanced to optimise the performance of optical communication systems.
To support bandwidth growth more efficiently, we need to expand the usable spectrum. This can be done by expanding into the L-band. Utilising the L-band doubles fibre capacity as we are adding an additional 4.8 THz of spectrum; however, it requires a whole new set of EDFAs for the L-band and a new set of transceivers that are L-band tunable, making it operationally and economically challenging.
An alternative path forward is to expand the utilisation of the spectrum in the C-band, leveraging the so-called Super C-band. Super C-band expands the usable spectrum from 4.8 THz to 6.1 THz. With commercially available amplifiers optimised for Super C and the latest 1.2 Tb/s-capable transceiver technology, this approach offers 27% more spectrum and up to 30% more fibre capacity – and all with lower power consumption and lower cost per THz of spectrum compared to the extended C-band spectrum.
Super C-band also postpones the need to expand into the L-band, thus reducing equipment, power, and operational costs. Further, a wider C-band operating spectrum enables wavelengths to operate at higher baud rates, which improves their reach and avoids or eliminates costly signal regeneration, which again improves fibre capacity.
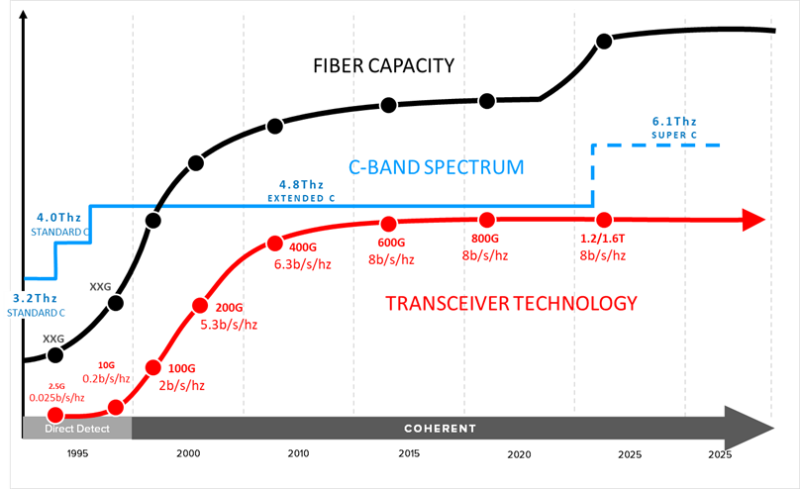
Additionally, Super L-band technology is emerging that adds 0.7 THz, increasing the available spectrum to 5.5 THz. Combined with Super C-Band, we now have commercially available access to 11.6 THz of spectrum, compared to the earlier 9.6 THz offered by extended C+L-band technology alone.
There are two approaches to further extend transmission capacity over silica-based optical fibres. One is spatially segmented or space-division multiplexing (SDM) technology represented by multicore fibres, and the other is the development of multi-band transmission using additional bands, including the O-, E-, S-, and U-bands beyond the C+L bands.
The most promising next transmission band for wide-area networking is the S-band, where the combination of S+C+L could almost triple fibre capacity compared to the C-band only. To get there, we need commercial S-band amplifiers based on thulium, rather than erbium, or we could use Raman amplification.
Today, O-band offers multiplexing of several wavelengths within its 1260-nm to 1360-nm spectrum and would require new types of amplification based on, for example, praseodymium-doped or bismuth-doped fibre amplifiers that would come with their own set of challenges.
Multicore fibres are currently proving their viability and provide significant improvements in increasing transmission capacity as they offer more cores within the same fibre for parallel data transmission.
A recent field trial conducted by researchers from Japan’s National Institute of Information and Communications Technology (NICT) demonstrated 319 Tb/s transmission using a four-core fibre combined with transmission in the S+C+L-bands over a stunning distance of 3,001 km.
The manufacturability and operational environment for these fibre technologies will continue to evolve before we see large-scale commercial deployments.
Another interesting development involves changing the transmission medium itself. Instead of transmitting signals in glass or silica as we do with today’s fibre optic cables, we could transmit wavelengths in air or a specific gas like nitrogen.
That’s exactly what hollow-core fibre (HCF) is all about. HCF eliminates the non-linear effects and chromatic dispersion inherent in today’s fibre-optic transmission solutions. With HCF, we can launch wavelengths with higher power across wider transmission bands and potentially open a path to higher modulation rates.
Moreover, hollow core fibre enables low-latency transmission and is gaining interest, especially in AI cluster connectivity in campus data centre interconnect. Manufacturing HCF in long lengths and overcoming operational complexities like fibre splicing and coupling hollow core fibres in commercial networks remain challenging and will require further advancements before mass commercial adoption is possible.
In summary, technological innovations in expanded transmission spectrum and new fibre types are on the way to cost-effectively support scalability and growth and improve efficiency in telecommunication networks.
Optical transmission technology is gaining more importance as it is by far the most economical medium to transmit large volumes of data over long distances with the highest capacity the lowest latency and with the lowest power consumption. In our all-connected world, this is a critical technology today.
RELATED STORIES
Nokia to acquire Infinera for $2.3bn
Infinera hires Regan MacPherson as chief legal officer and corporate secretary